As the Global Head of Marketing and Communications at Triton Hydrogen, I’ve become fascinated with exploring the complex challenges facing the hydrogen energy sector, especially those confronting engineers and companies striving to leverage hydrogen as a clean energy source.
Despite hydrogen’s clean energy promise, numerous obstacles, from material issues like embrittlement and permeation to broader concerns over cost, scalability, and safety, hamper its full potential. This article highlights these challenges, emphasising the critical role of material science in advancing the hydrogen economy. By addressing these hurdles, we underscore the importance of innovation and collaboration in overcoming the barriers to a sustainable hydrogen infrastructure. Read on to understand the fundamental problems and the path forward in the hydrogen sector.
Here are some of the main problems with hydrogen:
Hydrogen Embrittlement (HE)
Hydrogen atoms can diffuse into metals, weakening their atomic bonds and causing brittleness, cracks, and failure. This is a major concern for pipelines, storage tanks, and other components exposed to hydrogen gas. Engineers need to develop materials resistant to embrittlement or find ways to mitigate its effects.
Permeation
Due to its small size, hydrogen molecules can readily permeate through many materials, causing leakage and safety hazards. Designing barriers that effectively block hydrogen passage while maintaining other desired properties, like strength and ductility, is a significant challenge.
High-Temperature Performance
For some applications like fuel cells and turbines, materials need to perform reliably at high temperatures and pressures while exposed to hydrogen. Traditional materials often degrade under these conditions, requiring engineers to develop new alloys or composites with superior high-temperature stability.
Cost and Scalability
Implementing widespread hydrogen use often hinges on cost-effective materials. Currently, many promising materials are expensive or difficult to produce in large quantities. Finding alternatives that are affordable and scalable for mass production is crucial.
Complex Interactions in Different Applications
Hydrogen can interact with other materials in unexpected ways, leading to corrosion, embrittlement, or other issues. Understanding these interactions and predicting their impact on material performance is critical for choosing the right materials for specific applications.
Lack of Standardised Measures and Materials
The hydrogen sector is still evolving, and more standardisation of materials and testing methods is needed. This makes it challenging to compare different materials and select the best one for a particular use case. Developing standards and harmonising testing procedures is essential for the sector’s advancement.
Safety Considerations
As with any energy source, safety is paramount when dealing with hydrogen. Material science engineers need to develop materials that are not only high-performing but also inherently safe, minimising the risk of leaks, explosions, and other accidents.
Life Cycle Assessment
Ensuring a truly sustainable hydrogen economy requires considering the entire life cycle of materials, from production and use to end-of-life disposal. We need to develop processes and materials that minimise environmental impact throughout their life cycle.
Material Compatibility for Various Storage Methods
Each hydrogen storage method presents specific material challenges:
- Compressed hydrogen: Finding lightweight, high-pressure tanks resistant to HE and hydrogen permeation remains a challenge. Composite materials are promising but require further development.
- Liquid hydrogen: Cryogenic temperatures (-253°C) pose challenges for materials selection. Stainless steel is commonly used, but it is vital to understand its long-term behaviour and develop alternatives with improved thermal properties.
- Solid-state hydrides: While offering high storage capacity, some hydrides suffer from slow hydrogen absorption/desorption kinetics and potential degradation during cycling. Finding materials with optimal reversibility and stability is key.
Cost and Scalability
Developing and implementing new storage and pipeline materials must be economically feasible. Scaling up the production of promising candidates while maintaining affordability is crucial for widespread adoption.
Leak-proof Seals and Coatings
Minimising hydrogen leakage during storage and transportation is paramount for safety and efficiency. Developing reliable, long-lasting seals and coatings with minimal permeation has been a major challenge for the industry.
Infrastructure compatibility
Transitioning existing natural gas infrastructure to hydrogen presents material adaptation challenges. Upgrading or replacing pipelines and storage facilities requires materials compatible with both fuels.
Summing Up
It’s important to note that these challenges are interconnected. Progress in one area often impacts others. For example, solving HE in pipelines might involve materials unsuitable for storage methods. Therefore, a holistic approach considering the entire hydrogen value chain is necessary.
Material science research in these areas is actively ongoing, with promising advancements like advanced alloys, composite materials, and innovative coatings, like Tritonex and Tritonor, offering hope for overcoming these hurdles. Addressing these challenges will pave the way for a safe, efficient, and scalable hydrogen infrastructure, essential for realising its potential as a clean energy carrier.
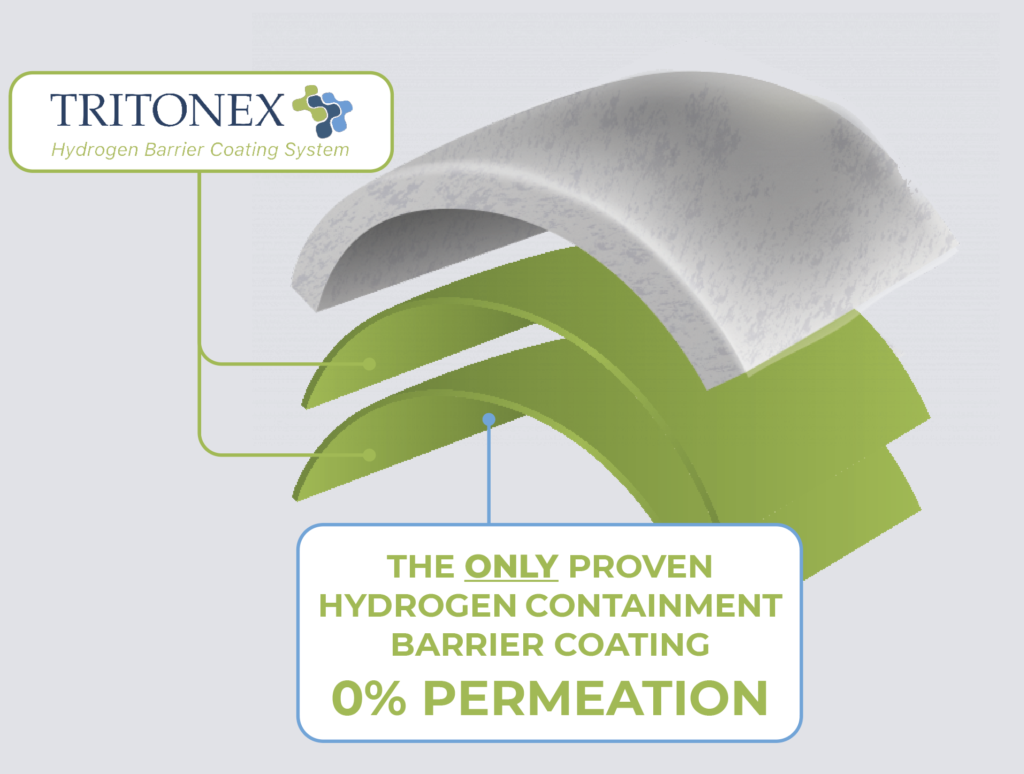